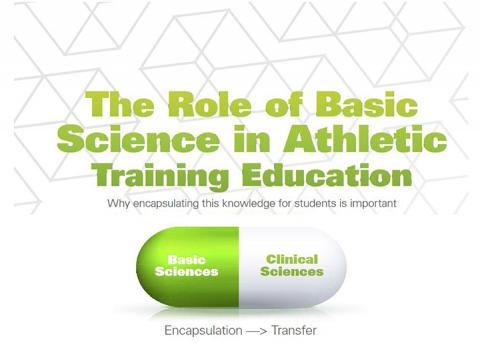
By Paul Geisler, EdD, ATC
Editor’s Note: This is an extended version of “The Role of Basic Science in Athletic Training Education” article in the April NATA News.
As most program administrators are undoubtedly aware, Standard 54 of the 2020 Standards for the Accreditation of Professional Athletic Training Programs calls for “prerequisite classes in biology, chemistry, physics, psychology, anatomy and physiology at the post-secondary level”.1, p. 10 The inclusion of “hard science” classes has long been a precedential practice in medicine, physical therapy and physician assistant education, based largely on the premise that they are critical to clinical development and professional acumen in those fields.
Although the working premise is “more science equates to better clinical scientists,” the actual impact prerequisite sciences have on professional education is an interesting topic of debate amongst health care educationalists. Given this longstanding practice in many health care programs, including some current athletic training programs, and acceptance of the new requirements for professional education, what can athletic training educators expect to glean from students taking these mandatory basic sciences credits before matriculating into the professional phase of their programs?
Basic Science in Medical Education
It was 1910 when William Flexner, a Baltimore high school science teacher, was appointed by the Carnegie Institute to study medical education in the Unites States, and come up with a critical plan for significant improvements and public buy-in.2 The standard “2 + 2” medical curriculum known by many today, demarcated by two years of isolated laboratory science foundation knowledge, followed by two years of clinical training can be traced to Flexner’s belief that rigorous immersion in the basic sciences were paramount for learning the clinical sciences and, in the end, improving patient care by producing better physicians. The structural implications from Flexner’s work influences many of today’s medical schools, though the AMA and progressive medical educationalists are working hard to implement more effective curricula, pedagogies and approaches to physician education (i.e., earlier patient exposures, competency based education, etc.).
Generally, the basic sciences in medical education consist of formal coursework in anatomy, physiology, genetics, pathophysiology, immunology, pharmacology and biostatistics, among others – intense and focused study of the foundational sciences considered necessary to provide a scientific platform for the subsequent clinical sciences and experiences. In contrast, medical education’s clinical sciences include more system-specific studies such as: cardiology, gastroenterology, neurology, psychiatry, dermatology and more. In theory, they are naturally, and automatically, connected and work together to deliver the bulk of the didactic curriculum in medical school, providing the necessary knowledge, theory and skills needed for competent clinical practice. But, as medical education researcher Nicole Woods points out in her 2007 review, there is actually “little basis for the assumption that basic science education is a critical part of medical training.”3
Outside of the core and related courses required by the Commission on Accreditation of Allied Health Education Programs (CAAHEP) pre-educational reform, athletic training has not demarcated specific categories of science in the pre- or professional curricula, nor has it required prerequisite coursework as part of accreditation compliance. But, current CAATE standards have chartered a more definitive direction with Section IV, Curricular Content, which has been partitioned into “prerequisite” classes (aforementioned Standard 54) and “foundational knowledge” (Standard 55).1 Standard 55 requires formal instruction in statistics, research, epidemiology, pathophysiology, biomechanics, exercise physiology, nutrition, human anatomy, pharmacology, public health and health care delivery/payor systems. Though not articulated as “required foundational knowledge” in Standard 55, other core athletic training content areas may be seen as equivalent to medicine’s clinical sciences by proxy – those focusing on orthopedic assessment, emergency or acute care, therapeutic modalities and exercise (intervention), EBP, general medicine and psychosocial health, etc. Regardless of their specific program or catalog course names, these core areas of study and application are, in effect, part of the clinical sciences that make up athletic training practice. On some level then, they must be supported by or integrated with other natural and social sciences and, thus, integral to the overall education of athletic trainers.
Numerous studies in medical education have discovered that basic science education in undergraduate education of physicians is a mixed bag, as far as how much bang for the buck they deliver to the end product – physician competence.4-8 Part of this mixed bag relates to the actual content (both depth and breadth), or the percentage (what is and what is not relevant) of basic science that’s fundamentally relevant to clinical practice. The other concern relates to the pedagogy of the basic sciences, or how they have been traditionally taught or delivered to bright and enthusiastic medical students. As renowned medical educator and scholar Geoffrey Norman states, “Effective instruction in the basic sciences requires specific pedagogical and assessment strategies that go beyond traditional ‘read and regurgitate’ approaches.” 9
On a positive note, basic science education in the first two years of medical school is believed to: 1) play a strong developmental role in helping students develop a conceptual framework for the subsequent learning of the clinical sciences (promoting or inculcating scientific thinking, weighing evidence, models of thought and systems-based approaches); 2) have an important, encoded role in clinical reasoning; and 3) play a role in clinical task performance, one beyond recall.3-9 On the other hand, those concerned with the large space that basic science education takes in an already dense, and challenging, medical curriculum, point out that 1) practicing physicians rarely use basic science for diagnostic reasoning; 2) a large portion of basic science content is irrelevant to actual medical practice; 3) rote memorization is too often the mechanism by which medical students “learn” required science knowledge (leading to poor retention and application); 4) accurate, basic science understanding often fails to help students explain clinical phenomena in great depth or detail; and 5) too great a percentage of the basic science knowledge base is not transferrable to other domains of learning or practice (due to the manner and timing with which they are taught).3-9 In other words, proponents of an updated, less Flexnerian medical education paradigm argue it takes more than two years of devotion to scientific facts, figures and rules to translate such hard earned wealth of scientific knowledge to clinical understanding, patient-centered application or professional expertise.
Encapsulating the Basic and the Clinical Sciences
It has been demonstrated that the integration and transfer of basic science principles and concepts into clinical contexts are far more important and meaningful than the pedantic ability for successful students to accurately recall facts, figures and laws learned in isolation years prior, out of context and without connectivity to clinical knowledge.3-9 What medical educators have repeatedly, and frustratingly, found is that they must often re-teach basic science facts that are, in fact, relevant to the medical or clinical knowledge under investigation, and they must do so in more enriched and meaningful manners. Clinical science faculty and clinical supervisors find they must purposefully connect the relevant biosciences to the clinical sciences in order for it to become significant for the student and, thus, to be of practical utility to the student clinician.
Henk Schmidt and Henny Boshuizen are Dutch medical educationalists, credited with putting forth the concept of encapsulation in 1992; a cognitive mechanism for “structuring knowledge” that Schmidt and Boshuizen express as vital for transitioning from intermediate to expert competence in medical practice.10 Simply put, encapsulation is a teaching/learning approach borne of the intentional marrying of relevant facts and figures from the basic sciences to germane principles and theories from the clinical sciences. Encapsulating basic and clinical sciences is a technique athletic training educationalists can leverage to help their students better acquire, link and make broader sense of what they are learning throughout the curriculum, and to then transfer that encapsulated knowledge into clinical practice and decision-making challenges. Further, effective encapsulation has been shown to improve recall accuracy and speed, diagnostic accuracy and problem representation – all hallmarks of adaptive expertise in medical practice.3
Encapsulation in Athletic Training: Examples
Knowledge from the world of physics concerning the electromagnetic spectrum is clearly fundamental to understanding many aspects of modality application and, thus, to clinical competence with such devices during patient care. But, can even the most capable professor of physics ensure athletic training students will learn such relevant knowledge in a manner that means anything to the student when he/she takes his/her therapeutic modalities course in the curriculum? Will that critical knowledge transfer from PHYS 101 to the clinical science of electrical modalities? Or, rather, will it be up to the athletic training educator to re-teach these relevant basic science concepts in the context of athletic training application and understanding?
It’s vital for athletic training students to understand how Newton’s force of gravity interplays with patellofemoral contact area, specific range of flexion, open and close kinetic chain actions of the tibiofemoral and patellofemoral joints, and resultant patellofemoral joint reaction forces in order to understand both the factors that contribute to patellofemoral pain syndrome to design effective intervention programs that are inclusive of those physics principles. Will learned knowledge of gravity’s forces transfer from PHYS 101 to an orthopedic assessment class without a mechanism for doing so?
There are also numerous examples from basic science courses fundamental to AT education and the core of what ATs do clinically, as well—anatomy and physiology. Despite the fact that athletic training students standardly complete and pass between four and eight credits of anatomy and physiology in the pre-professional phase, there are many athletic training educators who lament the amount of anatomy and physiology they still must teach, re-teach and integrate with their student (regardless of grades earned) over the remaining coursework – in the more advanced clinical sciences (i.e., orthopedic assessment) inherent in the latter, professional phase. Though most students may be able to recall the origin, insertion and action of the muscles of a certain joint when called upon for an exam or clinical inquiry, for example, it’s often the case that many can’t easily or effectively transfer that information into relevant clinical contexts without considerable struggle or assistance.
When a student can accurately recite that the hip abductor and external rotator muscles concentrically contract to abduct and externally rotate the femur, but can’t explain how they work eccentrically to control femoral adduction and internal rotation during the absorptive phase of gait or landing, it indicates a lack of transferability to the clinical context when assessing an athlete with iliotibial band impingement or patellofemoral pain syndrome. There are dozens of examples involving muscle function and dysfunction that would be much easier to teach and address in the clinical science classes if students knew more than the black-and-white facts and figures mastered in their anatomy and physiology coursework and if they had the relational knowledge structures that help link facts to function, improve recall and application and enhance clinical reasoning.
Although a student may be able to: recall and define the systolic and diastolic components of blood pressure; understand that blood pressure is a byproduct of cardiac output and total peripheral resistance; and indicate normal or ideal blood pressure readings, his/her failure to explain what happens to the blood pressure as a result of internal or external hemorrhaging indicates a failure to transfer those tenets of basic science to clinical relevance. When an athletic training student can’t explain the physiological aspects of hemodynamics, and can’t link together the systematic relationship between venous return, cardiac output and peripheral resistance as core to the explanation for a hemorrhaging patient with dramatic hypotension, it indicates that knowledge learned in physiology hasn’t been transferred to clinical practice.
Future Directions for Teaching and Scholarship in Athletic Training Education
In many instances, and often after the fact, it’s the athletic training educator who must do the difficult, but necessary, work of encapsulating the basic and clinical sciences for their athletic training students in order to promote and develop sound clinical reasoning skills. It is the effective class or clinical instructor who’s responsible for, and in an ideal position to: identify, integrate and link the contextually and clinically relevant bits of anatomy, physiology, chemistry and biology with the suitable features of the clinical sciences in athletic training, and those particular to our domains of practice and professional body of knowledge. Athletic training educators dedicated to the notion of knowledge structure and transferability should thus appreciate Schauber et al’s description of the negative relationship found between “early levels of bioscientific knowledge and subsequent gains in clinical knowledge” when encapsulation and transferability are not implemented.11 In short, the earlier basic sciences are introduced and the less encapsulated they are, the more they are lost; as clinical knowledge improves with increasing clinical experience, basic science knowledge diminishes.
To be fair, basic science professors are part of the medical faculty in medical schools, and are integral and beholden to the overall team approach to medical education. Non-AT faculty are not responsible for athletic training education outcomes, and it shouldn’t be up to our colleagues from the physics, biology and chemistry faculty to identify or encapsulate the requisite science facts from their fields into contextually relevant athletic training knowledge and skills. In the end, this is the responsibility and domain of the athletic training educationalist.
Currently, no research exists on science transferability in athletic training education, nor can sources that specify which science principles are fundamental to the clinical sciences or foundational knowledge of athletic training be identified. Given these realities, there is an incredible amount yet to discover about encapsulation and transfer in athletic training education and practice. As you design and advance your pedagogy and courses, think about which basic scientific principles relate to the clinical principles you are teaching, and look for and develop stimulating and effective sways to encapsulate that knowledge for and with your students – if the evidence from medical education carries over to athletic training (which it should), you might notice a greater level of transferability, more sophisticated clinical reasoning and decision making, and you just might improve your program’s educational outcomes along the way. Give it a try; go ahead and encapsulate.
References
- 2020 standards for the accreditation of professional athletic training programs. Master's degree programs. Commission on Accreditation of Athletic Training. https://caate.net/wp-content/uploads/2018/09/2020-Standards-for-Professi.... Accessed January 11, 2018.
- Duffy TP. The Flexner Report—100 years later. Yale J Biol Med. 2011;84(3):269-276.
- Woods NN, Brooks LR, Norman GR. The role of biomedical knowledge in diagnosis of difficult clinical cases. Adv Heal Sci Educ. 2007;12(4):417–426.
- Sibbald M, Neville A. A hundred years of basic science in medical education. Perspect Med Educ. 2016;5(3):136–137.
- Woods NN, Neville AJ, Levinson AJ, Howey EH, Oczkowski WJ, Norman GR. The value of basic science in clinical diagnosis. Acad Med. 2006;81(S10):124–S127.
- Norman G. Teaching basic science to optimize transfer. Med Teach. 2009;31(9):807–811.
- Woods NN, Brooks LR, Norman GR. It all make sense: biomedical knowledge, causal connections and memory in the novice diagnostician. Adv Heal Sci Educ. 2007;12(4):405–415.
- Mylopoulos M, Woods N. Preparing medical students for future learning using basic science instruction. Med Educ. 2014;48(7):667–673.
- Norman G. The basic role of basic science. Adv Heal Sci Educ. 2012;17(4):453–456.
- Schmidt HG, Boshuizen HPA (1992) Encapsulation of Biomedical Knowledge. In: Evans D.A., Patel V.L. (eds) Advanced Models of Cognition for Medical Training and Practice. NATO ASI Series (Series F: Computer and Systems Sciences), Vol 97. Springer, Berlin, Heidelberg, pp. 375-290.
- Schauber SK, Hecht M, Nouns ZM, Dettmer S. On the role of biomedical knowledge in the acquisition of clinical knowledge. Med Educ. 2013;47(12):1223–1235.